The DNA origami contains a scaffold and hundreds of staples. Each sequence requires different annealing temperatures. Beside annealing temperature, there are other folding conditions that will influence the final structure. For example, the concentration of MgCl2 will have a great impact on the stability of DNA. Different ratio of scaffold and staples will also cause distinct results.
​
In the beginning, we tested several concentrations of MgCl2 and several staple to scaffold ratios to find out the optimal condition.
​
We tested different concentrations of MgCl2 in folding process. According to the electrophoresis result shown in Figure H1 and Figure H2, the structures folded with 20mM and 25mM MgCl2 were stuck in the wells. 10mM and 15mM groups showed larger clear band than negative control.
These data implied that our monomer folding was successful because there was an obvious band shift in experimental groups. Nevertheless, the result also indicated that 20mM and 25mM groups were not ideal conditions since all the scaffolds and staples were trapped in the wells. This result was probably due to misfolding or undesired interactions between two monomers. We further compared the intensity of band in 10mM and 15mM groups, and the 10mM group was slightly stronger than 15mM group. As a result, we chose 10mM as the optimal concentration of MgCl2 in folding process.
​
The Optimization of Folding Condition
​
​
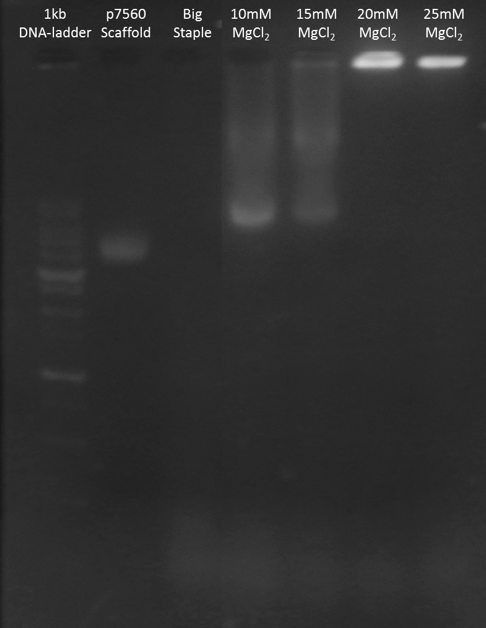
Figure H1. Big Monomer MgCl2 Test
​

Figure H2. Small Monomer MgCl2 Test
​
In order to find out the optimum proportion between scaffold and staple, we tested different ratios of scaffold and staple in folding process. According to Figure H3 and Figure H4, the band of complete monomers were almost all the same and the excess staples increased as the ratio get smaller. Because the result did not show any difference in three experimental groups, we chose scaffold : staple = 1 : 5 to fold our monomer.
​

Figure H3. Big Monomer Staple : Scaffold Ratio Test
​
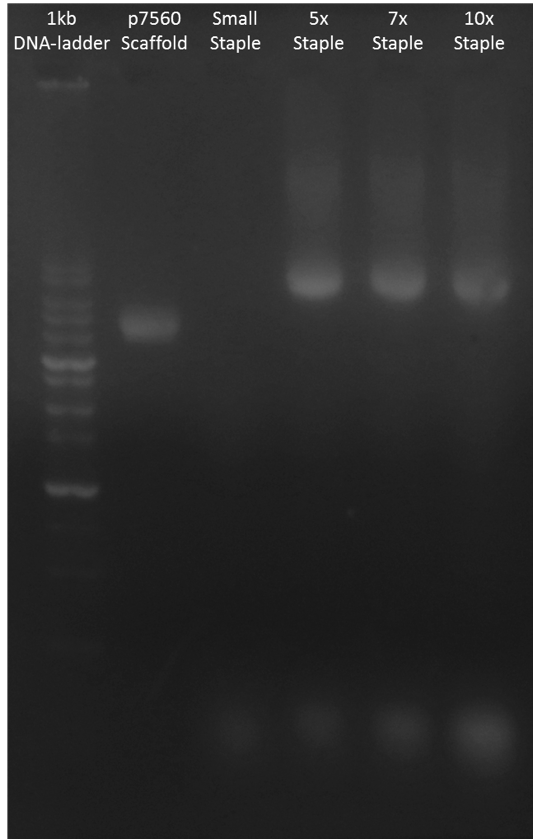
Figure H4. Small Monomer Staple : Scaffold Ratio Test
​
​
Although the electrophoresis data demonstrated that the monomers successfully folded due to the position of the major band. It was still possible that the structure was different from what we designed. For this reason, we tried to further identify the structure of the folded monomers by TEM.
Big monomer is shown in Figure I1, and Small monomer is shown in Figure I2. In these TEM images, we can see long, straight, needle-like rods with noticeable spots on both ends. Notably, one of the spots is larger than the other.
The shapes of the rods coincided with the expected shapes of our monomers. After comparing with the monomer model in caDNAno, we believed that the larger spot was the ring and that the littler spot wass the base of the designed structure.
Although most monomers turned up to be straight, there were still some wiggly monomers. This was because of the interplay of Browian motion and persistence length of the monomers.
The synthesized monomers often aggregated into clusters or "webs". The origin of aggregation might be certain unwanted assembly or non-specific interactions (such as van der Waals force) between NanoMuscles. For the clarity of explanation, we displayed here only the required TEM images of NanoMuscles.
​
Big and Small Monomer identified by TEM
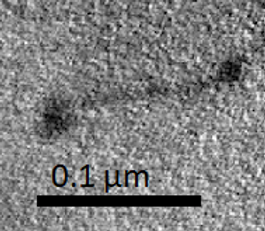
Figure I1. Big Monomer
​
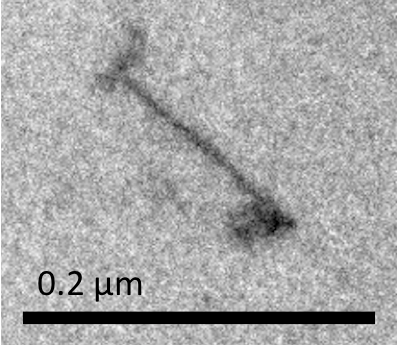
Figure I2. Small Monomer
​
​
After confirming the structure of monomers, we wanted to combine these two type of monomer. However, the excess staples in the sample might interfere with the following reactions. Therefore, we tried to purify two folded monomers.
The method we tried was ultracentrifugation which employed a column to keep the big structure and filters out the staples. To purify as clean as possible, we washed our product with buffer two times and collected the filtrate. After the whole process finished, we ran electrophoresis to find out the best condition.
In Figure J1, we could not see any band in the first and second filtrate. In the collection band, there was only one clear band which was slightly larger than the scaffold at the same position of the folded but unpurified monomer. This data indicated that the purification was successful because the staple disappeared and this process did not destroy the folded monomers.
The first filtrate contains nothing was probably because the original solution was diluted 2x before we did the purification. And the second filtrate was diluted by the buffer that we used to wash. So, the staple concentration was too low to be observed.
​
Purification
​
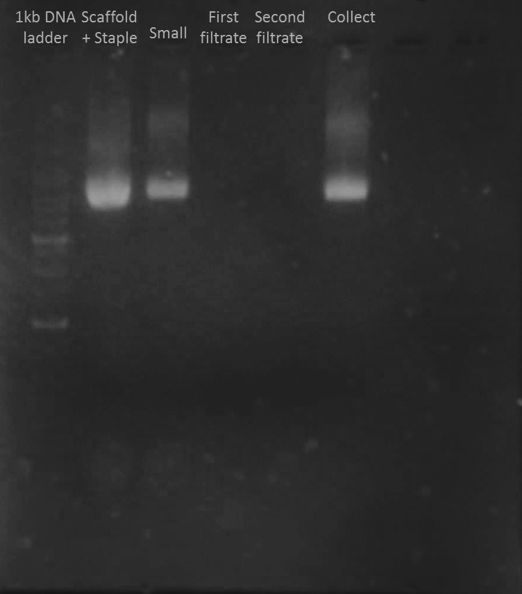
Figure J1. Purification Test
​
​
Big and Small monomers were combined by ten complementary base pairs, which was designed to be close to the rings. We named these complementary base pairs "dimer-combining sequences". The location of the dimer-combining sequences were designed to ensure that the axle would be in the ring of the other monomer when they combined.
NanoMuscle can stretch because there are two sets of complementary sequences on the ring and the axle. One of the sequences sets, which we named the "contraction sequence", lies on the ring of the Small monomer and the axle of the Big monomer near the base. The other sequences sets, which we named the "extension sequence", lies on the ring of the Small Monomer and the axle of the Big Monomer near the ring. In case the monomers anneal with each other in the wrong way, we blocked the extension and contraction sequences on the axle by two staples called "blocking staples" before combining the two monomers.
An observed “open Dimer” is shown in Figure K1. The Dimers are straight, and they are approximately twice the length of a single monomer. It is also noteworthy that the “larger spots” are aligned toward each other.
We designed that the Big and Small monomers attach to each other at their ring ends. As we expected, the larger rings were pointed to the middle of the Dimer. We also observed few Dimers that bent, or are even V-shaped. This was probably because of the flexible property of DNA origami or particular undesired combination of monomers.
​
Dimer(open)
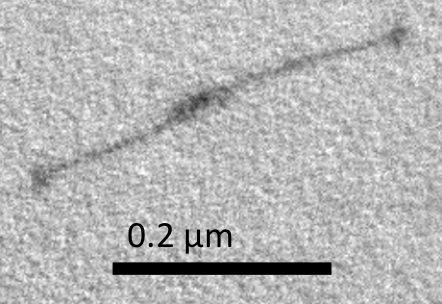
Figure K1. Dimer (open)
​
​
In the previous steps, the rings remained unclosed to allow the axle to get into the ring. Once the combination was completed, the next step was to close the ring of the "open Dimer". This made the Dimer stay in an interlocked configuration.
In our design, there are seven unpaired sequences at the edge of both sides. Each sequence contains ten base pairs. In order to close the ring, we added a complementary sequence, named ring-closing sequences, to connect both sides. The ring-closing step was completed when all the ring-closing sequences were annealed. We then obtained the "closed Dimer".
The observed "closed Dimer" is shown in Figure L1. The observed closed Dimer is also straight, with two large spots overlapping at the middle.
The results showed that the Dimer retained its configuration without observable conformational change after the ring-closing staples were added.
​
Dimer(closed)
​
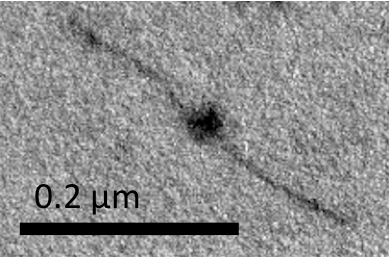
Figure L1. Dimer (closed)
​
​
After the rings were closed, the Big and Small monomers were locked to each other. In order to let our NanoMuscle move freely, we released the dimer-combining sequences which attached the Big monomer to the Small monomer.
In our design, there are ten extra unpaired base pairs in addition to the ten complementary base pairs of the dimer-combining sequence on the Big monomer. When we intended to detach two monomers, we added the "releasing staples" which contained twenty base pairs totally complementary to the Big monomer’s sequence. Since the Big monomer’s sequence had greater affinity to the releasing staples than the dimer-combining sequences on the Small monomer, the releasing staples substituted the dimer-combining sequences on Small monomer. Consequently, the releasing staples "released" the closed Dimer.
In this step, we obtained the interlocked "Free NanoMuscle". Since the blocking staples remained on the the axle of the Big monomer, the monomers moved freely along the axle of the other ones.
Figure M1 shows a long NanoMuscle with a similar configuration of the closed Dimer, and Figure M2 shows a medium-sized NanoMuscle in which two monomers partially overlap. Figure M3 illustrates a short NanoMuscle where the monomers totally overlap. In these three figures, the spots on the ends of the monomers all align in the direction of the NanoMuscle.
Three different configurations of NanoMuscle observed in TEM image indicated that “releasing staple” really released the closed Dimer as our expectation. Since the movement of NanoMuscle paralleled the direction of axle, we convinced that the interlocked structure of NanoMuscle was well-synthesized.
​
Free NanoMuscle
​
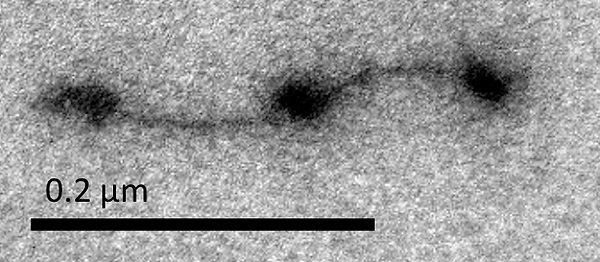
Figure M1. Long Free NanoMuscle
​
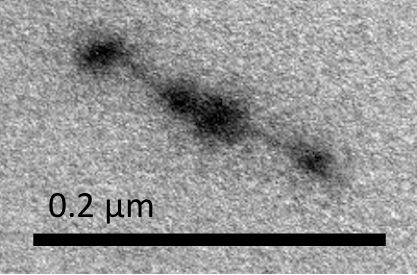
Figure M2. Medium Free NanoMuscle
​
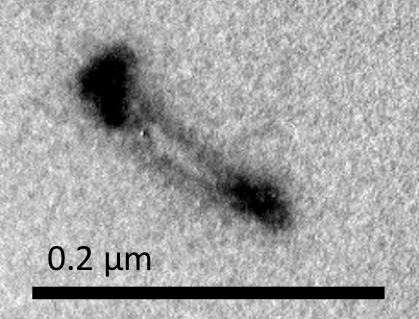
Figure M3. Short NanoMuscle
​
​
The movement of NanoMuscle requires the interaction between the ring of the Small monomer and the axle of the Big monomer. Their interaction is controlled by two sets of sequences: the extension sequences and the contraction sequences. These two sequences are previously blocked during the synthesis of interlocked NanoMuscle. To functionalize our NanoMuscle, we removed the blocking staples.
In order to remove the blocking staples, we added the anti-blocking staples. There are 30 complementary base pairs between the anti-blocking staples and the blocking staples, while there are only 20 base pairs complementary to the extension or contraction sequences on the monomers. Therefore, the anti-blocking staples removed the blocking staples from the Free NanoMuscle. We finally obtained the Complete NanoMuscle.
Figure N1 and Figure N2 are the observed Complete NanoMuscle. We can see NanoMuscle in its full length and NanoMuscle in its shortest length in these figures.
Both extended NanoMuscle and contracted NanoMuscle presented in our sample. However, the extended type was slightly more than the contracted type. This suggested that NanoMuscle preferred its extended configuration to the contracted configuration. In addition to these two configuration, one NanoMuscle with medium length was also observed in the sample. Nevertheless, since the extended and the contracted NanoMuscles outnumbered the medium-length NanoMuscles, we confirmed that the removal of blocking staples was completed.
​
Complete NanoMuscle
​
Figure N1. Long Complete NanoMuscle
​
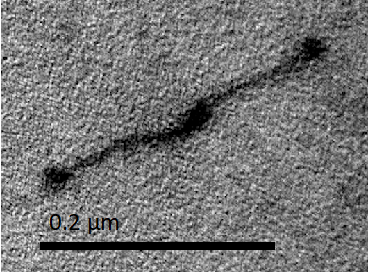
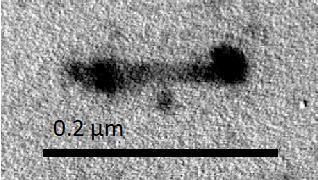
Figure N2. Short Complete NanoMuscle
​
​
In the following sections, we added “Fuel” and “Anti-fuel” to test the function of NanoMuscle.Single-stranded DNA “Anti-fuel” was added to the Complete NanoMuscle to test the extension of NanoMuscle. (For the details of NanoMuscle mechanism, please check out Functional Mechanism.)
Figure O1 are the Anti-fuel NanoMuscles. The Monomers of the NanoMuscles slightly overlapped. Many of the observed Anti-fuel NanoMuscle had a overlapping length shorter than the length in medium-length Free NanoMuscle, but longer than the length in Dimer.
​
The extension sequence was designed to be farther to the ring structure, compared to the dimer-combining sequence. So, the Monomers in Anti-fuel NanoMuscle was more overlapped than in Dimer. In this stage, most NanoMuscles appeared to be extended, so we confirmed that we could control the equilibrium configuration of NanoMuscle with Anti-fuel sequence.
​
Anti-fuel (extended) NanoMuscle
Figure O1. Antifuel (extended) NanoMuscles
​
​
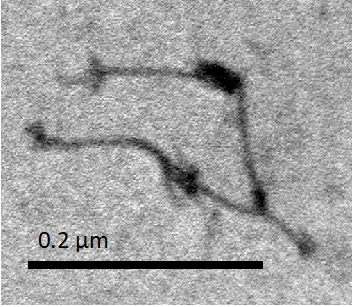
Single-stranded DNA “Fuel” was added to the Complete NanoMuscle. Thus, the NanoMuscle is expected to contract to its shortest length.
Figure P1 shows a contracted NanoMuscle. The total length of this NanoMuscle is near the length of a monomer. This Fuel NanoMuscle has a broader diameter than Monomers, and we can slightly distinguish the two different Monomers by the white line between the Monomers. Both of the spots at the ends were stained dark and wide.
Since the length of a Fuel NanoMuscle and a monomer were almost the same, it was hard to distinguish whether it was a contracted NanoMuscle or just a Monomer. However, it was obvious that the diameter of a contracted NanoMuscle was twice as the diameter of a single monomer in the same TEM image. Thus, we confirmed that after combining with the added Fuel sequences, the NanoMuscle contracted.
​
Fuel (contracted) NanoMuscle
​
Figure P1. Fuel (contracted) NanoMuscle
​
​

The extension and contraction of NanoMuscle are reversible. To test whether the reaction was reversible, we added excess contraction sequences after adding the extension sequences. We tried two procedures before we identified the result by TEM. One was that excess staples had been removed by ultra-centrifugation purification before the contraction sequences were added, and the other one was similar but without purification process.
The results shown in Figure Q1 and Q2 demonstrated that in both procedures, many contracted NanoMuscles were found. However, there were more extended NanoMuscles in the sample which was made without purification.
Many contracted NanoMuscles were observed after the two-step reaction. Therefore, we confirmed that the conformational change of our NanoMuscle wass reversible. Additionally, the results of the two procedures showed that our NanoMuscle had a better yield of the desired configuration with a purification step (which removed excess staples in the solution) between the two counteracting reactions.
​
Reversible Reaction of NanoMuscle
Figure Q1. Add Anti-fuel (extend) → Purify → Add Fuel (contract)
​
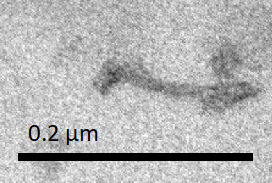
Figure Q2. Add Anti-fuel (extend) → Add Fuel (contract)
​
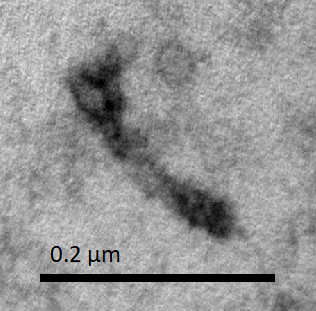
In conclusion, we successfully assembled the Big and Small monomers to form a functional NanoMuscle. The NanoMuscle is able to extend and contract by combining to Anti-fuel or Fuel sequences.
​
Since the DNA origami technique was created in 2006 [R-1], many different designs and applications of DNA origami were developed over these ten years, including drug delivery system, biosensor, bioreactor and so on. [R-2 to R-4] However, there was no study that created a nanomachine able to do the work such as contraction or extension. As a result, we created NanoMuscle.
Although one unit of NanoMuscle seems impossible to provide enough force to do variable applications, we expect that the NanoMuscles can be assembled in series or in parallel and turn into a “Macro-NanoMuscle”. In the future, NanoMuscle may be an important component which is responsible to do contraction or extension in nanomachines.
​
​
​
Citation:
[R-1] Rothemund, Paul WK. "Folding DNA to create nanoscale shapes and patterns." Nature 440.7082 (2006): 297-302.
​
[R-2] Douglas, Shawn M., Ido Bachelet, and George M. Church. "A logic-gated nanorobot for targeted transport of molecular payloads." Science 335.6070 (2012): 831-834.
​
[R-3] Pei, Hao, et al. "Scaffolded biosensors with designed DNA nanostructures." NPG Asia Materials 5.6 (2013): e51.
​
[R-4] Fu, Yanming, et al. "Single-step rapid assembly of DNA origami nanostructures for addressable nanoscale bioreactors." Journal of the American Chemical Society 135.2 (2012): 696-702.